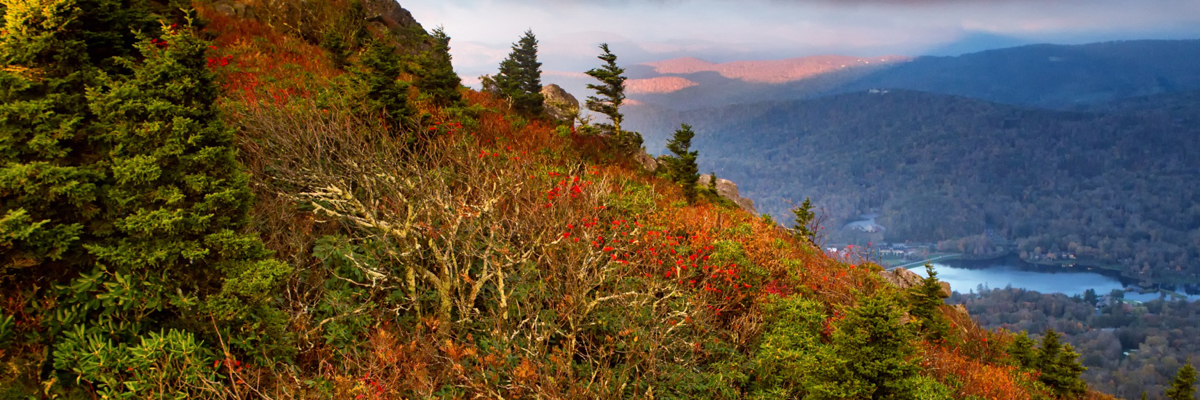
Introduction to the Situation
Check out this photo of a senescing oak leaf that I took last Saturday, October 8, 2010 along the Blue Ridge Parkway in western North Carolina. Note the unique coloration pattern whereby the veins have remained quite green, but the leaf blade has turned yellow. This is a common occurrence as leaves turn colors in the fall, yet is rarely commented upon, and, as we shall see, the mechanisms responsible for this pattern are still poorly understood.
Why Do Leaves Appear Green?
The green color in leaves results from the production of a compound called chlorophyll (chl). We see the leaf as green because this compound is most efficient in absorbing red and blue wavelengths of light, and relatively inefficient in absorbing the green wavelengths. This means that the light reflected from the leaf back to our eyes is enriched in the green spectrum, making it appear green to us.
There are Many Different Kinds of Chlorophyll
There are several different types of chl, each specific to a different class of organism. For example, there are six different bacteriochlorophylls, which are found only in bacteria. Brown algae have chl a and chl c, as do diatoms, but red algae contain chl a and chl d. Green algae, like all higher plants, contain chl a and chl b, with chl a being the most abundant form. In fact, one line of evidence that higher plants are evolutionarily descended from green algae, and not the red or brown algae, is the fact that both groups contain the same two forms of chl molecules. Chlorophyll molecules absorb various wavelengths of light which begins the process of converting solar energy into chemical energy, which is the basis for most of life on earth.
How Much Chlorophyll is Out There?
Each year, about 1.2 billion metric tons of chlorophyll are produced and degraded worldwide, with 75% of this amount occurring in aquatic ecosystems, even though visually, we see the ocean as blue, and the land green. This is because the small photosynthetic organisms in water generally have shorter life spans than land plants, resulting in much higher turnover rates (148 times as high in fact!).
The Synthesis and Breakdown of Chlorophyll can be Seen from Outer Space
This synthesis and degradation of chl is one of the most important biological events on earth, and easily observable from space. With satellite imagery we can easily see the earth green up in the summer, and turn brown in the winter. On a more local scale, we can even follow the fall leaf color front as it moves southward, by using high resolution images obtained by NASA and other governmental agencies. Based on these photos, it is estimated that fall colors tend to move south at rates between 28 and 47 miles per day, depending on longitude. At those speeds, the fall color front would move from the NC - VA border to the NC - GA border, a distance of about 150 miles directly north-south, in about 3-5 days, which is what we usually experience each year.
Why Green Veins and Yellow Leaf Blades?
It's time to get back to our original question of why leaf veins stay green longer than the leaf blade when leaves senesce in the fall. Obviously, chl is being lost from the leaf blade more than it is from the veins. The question we need to ask then, is how is this being accomplished, and why? Something must be happening to the relative rates of synthesis and degradation in the leaf blade that differ from that in the veins.
Plants That Grow on Alkaline Soils can Develop Interveinal Chlorosis
Plants that grow on calcareous soils (those high in calcium, with a high pH, i.e., alkaline soils) often suffer from the phenomenon which we call interveinal chlorosis, or IVC. Interveinal means between the veins, and chlorosis means a lack of chlorophyll. Often, leaves that lack chlorophyll are yellow. The yellow comes from accessory pigments in the leaf called carotenoids, which assist chl molecules in the process of capturing light. They also help dissipate excess light energy if too much light strikes the leaf. These pigments are often yellow, which is why a leaf with IVC appears yellow between the veins.
So, why does growing on calcareous soil induce the phenomenon of IVC? Soils that are alkaline and have a high pH prevent the absorption and metabolism of iron (Fe). Iron becomes insoluble at high pH, and plants have trouble taking it up from the soil. Iron is a necessary nutrient for plants and required for the plant to synthesize chl. Without it, the plant will become chlorotic, mainly on the new leaves, since Fe is not very mobile within the plant.
To Understand IVC Requires a Lesson on the Structure of a Chlorophyll Molecule
The figure below shows the chemical structure of a chlorophyll molecule. While it may look very complicated, it is actually fairly simply constructed. The bottom part, which looks like a long tail, is used just like it was a tail—to hold the molecule in place on the membranes inside of a chloroplast. A chloroplast is a small structure about the size of a bacterium in a leaf cell in which the chl is contained and where the process of photosynthesis occurs.
The top part of the chl molecule has a ring of carbon and hydrogen containing units, and on the inside there are four atoms of nitrogen (N), to which is bonded one magnesium (Mg) atom.
What do We Mean by OIL RIG?
It is the Mg atom that donates an electron to another molecule when light strikes the chl. When a photon (a packet of light energy in the quantum sense) strikes the chl molecule, it adds its energy to the molecule, causing an electron on the Mg atom to jump to a higher energy state. This is a very unstable condition, and if another molecule is nearby, that electron can be grabbed by that molecule. When one molecule accepts an electron from another molecule, it is said to be reduced. If it gives one up, it is said to be oxidized. One way to remember which is whch is to use the phrase OIL RIG, which stands for Oxidation is Loss, Reduction is Gain.
In a leaf, there are a series of molecules that transfer electrons (these are called oxidation-reduction reactions) until they are finally captured at the end of the electron transport chain in a molecule known as NADPH. This molecule is eventually used to make sugars in another part of the photosynthetic process, something we will not get into in this essay.
Where does the Oxygen come from in Photosynthesis?
Meanwhile, our chl molecule is still missing an electron, which is also a very unstable state. The only way to get back to the original state prior to absorbing that photon is to replace the missing electron. But where can the leaf find a ready source of available electrons? Turns out that water is a great source of electrons, but only if you can split it apart into its components of hydrogen and oxygen. And leaves have developed a powerful molecular water splitting complex that can achieve just that. Once the missing electron is replaced by one from water, the chl is ready to accept its next photon. By removing the two H from the water molecule, free oxygen is liberated. This is how plants provide much of the oxygen with which you and I breathe every day. So, don't forget to thank a plant for the work they do to make your life possible!
What does Iron have to do with all this?
But prior to all this light absorbing and photosynthesis, the leaf had to make the chl molecule from scratch. In one of the last stages in this process, an Fe atom is inserted into the top section of the chl to hold a place for the Mg atom that will be added later. If the leaf can't access enough Fe, then it also can't make chl molecules, and it will appear chlorotic.
Now We Finally Get to Interveinal Chlorosis and Fall Leaf Colors!
Based on what we know about chl and Fe metabolism, this suggests that perhaps as these oak leaves senesce in the fall, their Fe metabolism goes awry, and the ability to synthesize new chl molecules is diminished. This means that degradation processes dominant over synthetic processes: more chl is degraded than made, and the amount of chl in the leaf declines over time. Remember, the amount of chl in a leaf at any one time is a function of how rapidly it is made, compared to how rapidly it is destroyed, i.e., it is dependent on the turnover rate. When the rate at which it is made is greatly reduced, but the rate at which it is destroyed remains the same, then the amount in the leaf will go down. This is the same as if you deposited money in your bank at a rate of $1/day, but withdrew $2/day. Eventually, your bank account will drop to zero!
But why do Degradation Processes Dominate in the Fall, and in Particular, in the Leaf Blade?
One answer may have to do with the fact that as leaves senesce in the fall, they begin withdrawing nutrients back into their stems (a process called retranslocation) so they can use them next year when the new leaves come out. This creates nutrient deficiency conditions in the leaf. Studies have shown that in citrus leaves, if the Mg, Zn, or K content is depleted (and these nutrients are among those that are commonly retranslocated) that this causes IVC. Perhaps the veins remain green because these nutrients are traveling through them on their way out of the leaf, which keeps the concentrations high enough to avoid chlorosis in the cells surrounding those veins.
Maybe Leaves Just Say NO
Another study implicates the compound nitric oxide (NO) in IVC. The authors of this study found that NO may be involved in the regulation of the oxidative state of the leaf, and that it might function as an anti-oxidant. As chl is degraded, it can become dangerous to the leaf, because if light strikes the partially degraded molecule, it can create reactive oxygen species (called ROS), such as hydrogen peroxide (H2O2), or something even nastier called superoxide (oxygen with an extra electron: O2-), among others, which can severely damage cellular membranes, resulting in the death of leaf cells. In this particular study, NO was able to donate an electron to an Fe atom (that is, reduce it!). This converted the Fe atom into a form that the leaf could take up and use to make chl. So, we could speculate that if there is more NO surrounding the veins, then the veins will stay green, whereas the leaf blade will bleach yellow.
Nitrogen Nutrition May be Involved Also
Part of this effect could also arise from differences in the pH between the veins and the leaf blade. In a study of sunflower plants, researchers found that plants fed high levels of nitrate (NO3-) developed IVC. When leaves are given NO3- as their sole source of N, they can only move it into their cells if they also move in a hydrogen ion (H+). When that happens, it removes these ions from the spaces outside the cells, which makes that area alkaline. Under these conditions the leaf cannot move Fe into its cells. This is because the Fe is oxidized (Fe+3, which is rust by the way), and plants can only move reduced Fe (Fe+2) into their cells. The area around the leaf veins has a lower pH, and so these cells can metabolize Fe more readily, and they retain their chl and remain green. But the pH in the leaf blade is higher (more alkaline) which interferes with Fe metabolism, and chl synthesis, so this portion of the leaf becomes chlorotic.
So, does that mean that leaves in the fall are suffering from NO3- induced IVC? Maybe, maybe not. These same researchers also found that mature leaves did not exhibit this response, but since they did not check senescing leaves, it is still possible that changes in leaf metabolism during the fall, especially with respect to NO3- nutrition, could be a factor also in causing IVC.
Conclusion: Nothing is Ever as Simple as it Looks!
Considering how long it took me to explain this, you can see that the simple pattern of green veins and yellow leaf blades has a very complicated origin, one that is not yet fully understood. Mineral nutrition, pH, retranslocation, turnover rates, hormones and other factors are all involved.
In closing, I'd like to emphasize that while birth and development are highly ordered processes, so too are the phenomena of senescence and death. When leaves begin to die in the fall, environmental and physiological cues result in genes being turned on in a highly regulated manner, biochemical reactions proceeding in a sophisticated and orderly sequence, resulting in the destruction of chl in a way that minimizes damage to the leaf. The adaptive result is that the leaf can recover vital nutrients and other useful metabolites before it falls off the tree, to be used to assist in the growth of next year's leaves. And when you think about all this, you have to admit that even death can be a beautiful journey if you just take the time to appreciate it in all its complexities. After all, death is a part of life, is it not?